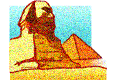 |
Protein modification by a glycolipid, glycosylphosphatidylinositol (GPI), is a conserved post-translational modification in eukaryotes. In mammals, more than 150 proteins, including receptors, adhesion molecules, hydrolases, and prions, exist as GPI-anchored proteins (GPI-APs) on the cell surface. The GPI biosynthesis and transfer to proteins take place on the endoplasmic reticulum (ER) membrane. The GPI-APs thus formed are then transported to the cell surface via the Golgi apparatus. During this process, the GPI anchors get remodeled (Fig. 1). Here, the structural changes undergone by GPI anchors in mammalian cells were summarized (1).
In the ER, two GPI remodeling reactions are carried out. First, a fatty acid (mainly palmitic acid) attached via an ester bond to the hydroxyl group at the 2-position of the inositol in PI is removed (Fig. 2), which is mediated by PGAP1, a GPI inositol deacylase. Bacterial PI-specific phospholipase C (PI-PLC) is known to cleave inositol-deacylated GPI anchors, but not inositol-acylated GPIs. For example, since GPI inositol is not deacylated in erythrocytes, the GPI-APs are not cleaved by PI-PLC. Subsequently, a side-chain ethanolamine-phosphate attached to the 6-position of the second mannose in GPI is removed by PGAP5 (Fig. 2). The conformationally changed GPI anchors are loaded into transport vesicles after being recognized by p24 family proteins, serving as cargo receptors for GPI-APs. Thus, these two reactions that take place in the ER are prerequisite for the efficient transport of GPI-APs to the Golgi apparatus (2).
In the Golgi, the GPI anchors first undergo lipid remodeling and then the addition of a glycan side-chain (Fig. 2). GPI is biosynthesized from PI, having an unsaturated fatty acid, such as arachidonic acid (C20:4), at sn-2 of the glycerolipid moiety. GPI fatty acid remodeling replaces the unsaturated fatty acid with the saturated stearic acid (C18:0) (Fig. 1). This reaction is initiated by the removal of the unsaturated fatty acid by PGAP3, the GPI-specific phospholipase A2. Subsequently, PGAP2 transfers a saturated fatty acid to the sn-2 position in GPI lipids. This GPI fatty acid remodeling is required for GPI-APs to associate with membrane microdomains called lipid rafts (3). On the other hand, GPI anchors that retain their inositol acyl-chain do not undergo this remodeling.
The addition of a GPI-glycan side-chain is initiated by the transfer of an N-acetylgalactosamine (GalNAc) moiety to the first mannose via a β1,4 bond (Fig. 2). This reaction is mediated by PGAP4, a glycosyltransferase localized to the Golgi apparatus. A β1,3-linked Gal can be added to the GalNAc residue, mediated by B3GALT4. Originally known as GM1 synthase, B3GALT4 is a glycosyltransferase that adds β1,3-Gal to GalNAc residues of glycolipid gangliosides such as GM2 and GD2. Interestingly, lactosylceramide promotes the addition of Gal to the GPI-glycan side-chain, but not GM1 synthesis (4). The precise mechanism by which glycosyltransferase distinguishes between the two substrates needs to be elucidated. Some GPI-glycan side-chains are further modified with sialic acid (Neu5Ac) on Gal. Analysis of the GPI structure of prions reveals that Neu5Ac modifies Gal via the α2,3 bond (5).
Here, I have reviewed the conformational changes that the GPI anchor undergoes after its addition to proteins. All these reactions do not occur uniformly, resulting in microheterogeneities in the GPI anchor structure depending on the cell type and protein species. In addition, other heterogeneities in mammalian GPI-lipids (existence of both 1-alkyl-2-acylglycerol and diacylglycerol types) and glycans (addition of the fourth Man) may originate during GPI biosynthesis in the ER (Fig. 1). Future analysis will clarify the regulatory mechanisms governing structural remodeling and the functional differences that arise due to the structural differences.
Figure 1.
Mammalian glycosylphosphatidylinositol (GPI) anchor structure. Mammalian GPI core structures are highlighted in pink. Variable structures are denoted with +/-.
|
Figure 2.
Structural changes to the GPI anchor in mammalian cells. The proteins involved in each reaction are shown below the arrows. GPI-TA, GPI transamidase.
Morihisa Fujita (Institute for Glyco-core Research (iGCORE), Gifu University)
References |
(1) |
Liu YS, Fujita M: Mammalian GPI-anchor modifications and the enzymes involved. Biochem. Soc. Trans. 48, 1129-1138, 2020 |
(2) |
Fujita M, Watanabe R, Jaensch N, Romanova-Michaelides M, Satoh T, Kato M, Riezman H, Yamaguchi Y, Maeda Y, Kinoshita T: Sorting of GPI-anchored proteins into ER exit sites by p24 proteins is dependent on remodeled GPI. J. Cell Biol. 194, 61-75, 2011 |
(3) |
Fujita M, Kinoshita T: GPI-anchor remodeling: potential functions of GPI-anchors in intracellular trafficking and membrane dynamics Biochim. Biophys. Acta. 1821, 1050-1058, 2012 |
(4) |
Wang Y, Maeda Y, Liu YS, Takada Y, Ninomiya A, Hirata T, Fujita M, Murakami Y, Kinoshita T: Cross-talks of glycosylphosphatidylinositol biosynthesis with glycosphingolipid biosynthesis and ER-associated degradation. Nat. Commun. 11, 860, 2020 |
(5) |
Kobayashi A, Hirata T, Nishikaze T, Ninomiya A, Maki Y, Takada Y, Kitamoto T, Kinoshita T: α2,3 linkage of sialic acid to a GPI anchor and an unpredicted GPI attachment site in human prion protein. J. Biol. Chem. 295, 7789-7798, 2020 |
Jun. 15, 2023
|