|
Chemical Evolution and Biological Recognition of Glycans
|
|
|
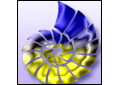 |
There are three reasons why we study glycans. First, they play an important role in living organisms (functional importance). Second, compared with nucleic acids and proteins they are substantially more difficult to synthesize and characterize, which, once accomplished, should therefore have the potential to introduce a new paradigm in life science (an attractive and challenging target for scientists). Third, the origin of glycans is closely linked to the origin of life and its evolution (relationship with the origin of life and its evolution) although this cannot be verified by experiments. If the origin of glycans is as old as or older than that of nucleic acids and proteins, proteins which are associated with glycosyltransferases and sugar-nucleotide syntheses (synthesis systems of glycans), and recognition systems of glycans (lectins, cytokines and antibodies against glycans, etc.), which specifically recognize and identify glycans derived thereof, can be assumed to have evolved in conjunction with each other (Fig. 1). |
|
|
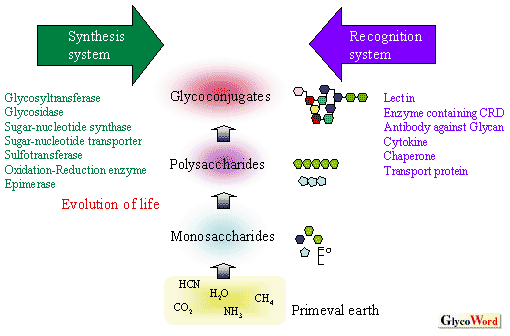 |
|
Fig.1. Synthesis system and recognition system of glycans.
The two systems have complemented each other from the early stages of life, and have evolved closely linked to the diversification and complexity of life. |
|
|
|
|
|
|
The topic, Comparative glycomics and life evolution comprises Glycans in various organisms, Evolution of glycosyltransferases and Evolution of lectins, and elucidates a variety of biological activities from the viewpoint of origin and evolution of glycans or comparative glycomics. Experts in these fields will present focusing on topical key terms. Here, the chemical evolution of glycans, the major promise on which this field is based, is discussed.
The theory (or hypothesis) on the origin and chemical evolution of glycans, developed by the present author (2), is summarized as follows. Some sacharides are assumed to have evolved chemically prior to the beginning of life due to the fact that they are synthesized nonbiologically (formose reaction, aldol condensation, and Lobry de Bruyn transformation, Fig. 2). Fructose, glucose, and mannose (the first triplet saccharides of the origin of life) that had been accumulated during the chemical evolution provided the materials for the origin of life. Advancing into the era of biological evolution, primeval forms of life took full advantage of the first triplet saccharides and developed diverse metabolic systems, producing a variety of late-comer saccharides (bricolage by biological evolution). Life forms took the most effective path of least resistance (e.g., frequent use of 4-keto-intermediates and aldol condensation), where glycans that are satisfactorily stable in the chair conformation of the pyranose ring were preferentially produced instead of all potential saccharides (aldohexoses). The stability of each glycan can be detrermined by the extent of the 1,3-diaxial interaction that destabilizes the pyranose-ring structure (Fig. 3). Galactose, which plays a central role in molecular recognition among the higher animals, cannot be synthesized by the first-triplet scheme of Fig. 2 (3-ketose is essential for galactose to be synthesized by the Lobry de Bruyn transformation). Galactose is assumed, therefore, to be a late-comer sugar that appeared at a latter stage of evolution (galactoses late-comer theory). Fructose, glucose and mannose are mutually transformed through 6-phosphorylated linear chains, whereas galactose is synthesized biologically through 4-keto-4-epimerization on UDP-Glc coenzymed by NAD+. The former takes place chemically without enzymes (Lobry de Bruyn transformation), and the latter does not take place without enzymes and coenzymes, indicating that the latter is fundamentally distinct from the former.
|
|
|
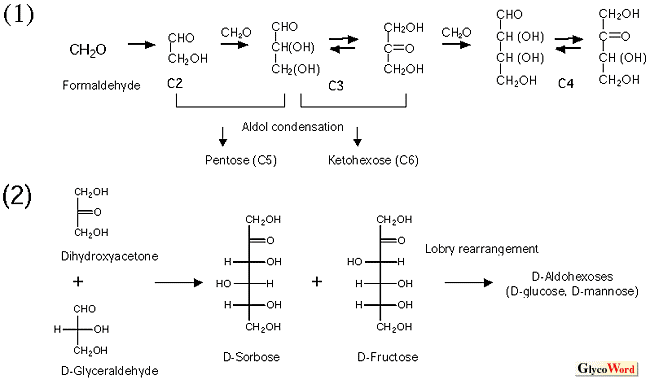 |
|
Fig.2. Schematics showing the chemical evolution of sacharides, assuming the formose reaction followed by aldol condensation and Lobry de Bruyn transformation.
(1) Self-polymerization takes place under basic catalysts from C1 compounds, such as formaldehyde, to C2, C3, C4,
, where C5 and C6 compounds may be synthesized by aldol condensation among C2 and C3 compounds. (2) When dihydroxyacetone and gryceraldehyde (both C3 compounds) react by aldol condensation under basic catalysts, sorbose and fructose are mainly produced, as reported by Fisher et al.. Aldol condensation between the trioses never produce aldose (glucose, etc.) due to its reaction mechanism. |
|
|
|
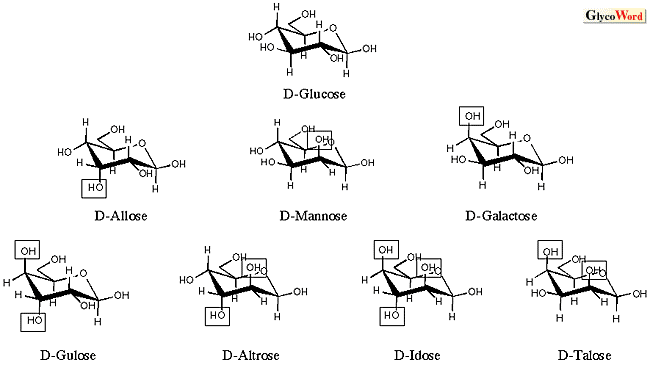 |
|
Fig.3. C1 chair conformation of D-aldohexose and orientation of axial hydroxyl groups.
Anomeric hydroxyl groups are unified in types. The axial hydroxyl groups that have the 1,3-diaxial interaction are enclosed by rectangles. Aldohexose (glucose, mannose and galactose) that has been preferentially selected by nature is shown to have the lowest energy in the 1,3-diaxial interaction. |
|
|
|
Glycans, which are assumed to have been first synthesized in the form of simple homo-polysaccharides (amylose, cellulose, etc.), are understood to have evolved into more complex hetero-polysaccharides (Evolution of the synthesis systems of glycans, see Glycogene). This evolution is assumed to have triggered the advent of proteins (lectins, see Lectin) related to the recognition system of glycans that recognizes each structure, identifies molecules, introduces biological signaling and facilitates infections. The synthesis system and the recognition system of glycans depend on each other and are still considered to be undergoing coevolution.
|
|
|
|
Jun Hirabayashi ( Research Center for Glycoscience, National Institute of Advanced Industrial Science and Technology (AIST) ) |
|
|
|
|
|
References |
(1) |
Miller SL: Which organic compounds could have occurred on the prebiotic earth? Cold Spring Harbor Symp. Quant. Biol. 52, 17-27, 1987 |
|
(2) |
Hirabayashi J: On the origin of elementary hexoses, Quart. Rev. Biol. 71, 365-380, 1996 |
|
(3) |
Taylor ME, Drickamer K: Introduction to Glycobiology. Oxford University Press (Oxford), 2003 |
|
|
|
|
|
Jul. 21, 2004 |
|
|
|
|
|
|